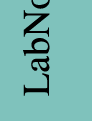

The bacterium Wolbachia within the cytoplasm of the ovary of its insect host (the wasp Nasonia vitripennis)
To harm or benefit? That is the question.
Bacteria show an amazing versatility in the ways that they interact with their hosts, and yet the number and kinds of genes, in effect the genetic recipes, that underlie this versatility remain a mystery. To determine these recipes, Seth Bordenstein, a postdoc in Jen's lab, is comparing genomes of closely related bacteria that have different effects on their hosts. He is doing so with an abundant group of bacteria, Wolbachia, that occur inside the cells of millions of insect species and nearly all infectious nematodes.
The Wolbachia inside the cells of nematodes are beneficial (i.e., mutualistic) to the worm and required for fertility. However, the Wolbachia inside the cells of insects, mites, and spiders are not required by the host; rather, they selfishly alter the ways in which offspring are produced. For example in some species, the Wolbachia can mysteriously convert male embryos into functional females, so that nearly all-female families are produced. Thus the effects that these bacteria have on their various hosts could not be more different. Yet the genomes of these Wolbachia are quite similar - in fact, similar enough to uncover what new genetic ingredients turn one genome into a recipe for mutualism and another into a recipe for parasitism.
Bordenstein is currently engaged in characterizing the disparity in gene content, organization, and transmission between these Wolbachia to reconstruct the pathway(s) by which some bacteria harm and others help their hosts. Wolbachia also have important implications for human health. It was recently discovered that Wolbachia produces the compounds that allow certain nematodes to cause river blindness, elephantiasis, and other debilitating tropical diseases. Understanding genome variation in this symbiont may provide insights into pathogenesis and lead to novel approaches for controlling these diseases.
|
 |
Return to Table of Contents
Living and Evolving Together
Bay Paul Center scientists explore complex partnerships of insects and their bacteria
In the world of evolutionary biology, survival of the fittest is a mantra often used to describe how species adapt and change over time. All living things face a relentless competition to survive and reproduce. The winners are the individuals that manage to pass their genes on to future generations, and any mutations that undermine an organisms ability to compete are usually weeded out of the gene pool.
This process of natural selection, however, is certainly not the sole mechanism responsible for shaping genetic evolution. Dr. Jennifer Wernegreen, Assistant Scientist at the Josephine Bay Paul Center for Comparative Molecular Biology at the Marine Biological Laboratory, is interested in better understanding how and why bacteria evolve the way they do. Bacteria species display an incredible amount of variation in the size, composition, and architecture of their genomes, but little is known about the evolutionary mechanisms that have given rise to this diversity. By combining molecular and comparative techniques that are the hallmarks of research at the Bay Paul Center, Wernegreen hopes to identify patterns and processes that control the evolution of bacterial genomes.
Wernegreens project is specifically designed to look at how intracellular bacteria have adapted to whats known as obligate symbiotic relationships with insect hosts. Millions of years ago, free living bacteria developed a close intracellular relationship with several species of insects, such as carpenter ants, aphids, tsetse flies, and many others. No one knows quite how the bacteria started living inside the insect cells, but once they were established there, they quickly made themselves indispensable. The bacteria are housed within the insect in specialized cells called bacteriocytes, and they earn their living by providing their host with services specially adapted for the relationship. For example, many species of insects rely on their bacterial symbionts for the production of essential nutrients that the insect is not capable of making on its own and cannot obtain from its diet.
 |
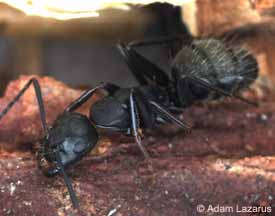 |
The ant, Camponotus pennsylvanicus
|
In turn, the bacteria have become completely dependent on their insect hosts and are no longer capable of surviving outside the host cell. As the bacteria have adapted to these relationships, known as obligate symbiosis, they have evolved highly streamlined genomes that are specialized to provide the host-beneficial functions necessary to support their symbiotic lifestyle. In the process, they have tossed out many of their genes5 out of 6 in the case of Buchnera aphidicola, the bacterial symbiont of aphids.
But why would a species discard so many of its genes? In organisms with small genomes, such as bacteria, losing genes usually means losing the ability to do something. Perhaps in the relatively static environment of a host cell, bacteria no longer needed to perform many of the functions necessary in free-living bacteria. Buchnera, for example, has lost many of the genes that encode cell membrane proteins. If the bacteria are well enough protected inside the bacteriocytes, then the loss of some cell membrane functions may not compromise their ability to survive. Thus genes encoding such superfluous functions would not be missed
when lost.
Another mechanism that plays a key role in the evolution of the small genomes, however, is genetic drift a process by which changes in gene frequencies are fixed merely by random chance. This drift is the result of a population bottleneck that occurs as bacteria are transmitted through insect host lineages. Because bacteria are passed directly from mother to offspring, the bacteria present in each successive generation represent only a small portion of the maternal bacterial population. There is no opportunity for these bacteria to recombine with genes from other populations, so when a gene is lost in the transfer, there is no chance of getting it back. And unless the lost gene is one that provides a vital host function (in which case the host would die and effectively eliminate the lineage), non-adaptive deletions and mutations will be preserved and passed on to future generations.
As a result of this process of genetic drift, most symbiotic bacteria have lost genes encoding DNA repair enzymes, and therefore have a limited ability to repair mutations in their DNA. Their genomes are also characterized by a disproportionately high content of A-T (adenine and thymine) base pairs, a feature that is likely the result of a strong mutational bias in favor of the G-C (guanine and cytosine) base pairs mutating to A-T pairs. This mutational pressure has caused degradation in the bacterial genome that has decreased the stability of their proteins.
In essence, symbiotic bacteria are living a lifestyle that results in a sort of genetic downward spiral, and they are often characterized as having limited evolutionary potential. Yet this lifestyle is one that has been successful for millions of years. Wernegreen says that the symbionts face a kind of paradox in that they are highly specialized and critical for the host, but all evidence points to their being plagued by harmful effects of mutational biases and genetic drift.
To better understand the balance between processes of natural selection, genetic drift, and mutational bias, Wernegreens lab is exploring the forces that drive and constrain genome reduction in endosymbionts. They are currently focusing on the genomes of several lineages of Candidatus Blochmannia, the bacterial symbiont of carpenter ants. They will also be comparing the Blochmannia genome to that of Buchnera, the bacterial symbiont of aphids, and Wigglesworthia, the symbiont of tsetse flies. These bacteria all evolved very similar relationships with their hosts, but they did so independently. Those genes that have been consistently retained across species are the most likely candidates for host-beneficial genes that have been preserved through natural selection.
 |
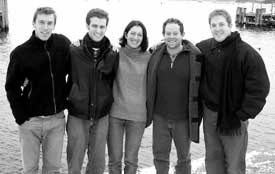 |
Wernegreen lab members: Josh Herbeck, Patrick Degnan, Jennifer Wernegreen, Adam Lazarus,
and Seth Bordenstein
|
These symbiotic bacteria are also very closely related to the free-living bacteria Escherichia coli. Millions of years ago, before the symbionts began shedding genes and adapting to an intracellular lifestyle, these bacteria would have had a very similar genome. Thus the modern genome of symbiotic bacteria represents a subset of the genes found in E. coli. By comparing the genomes of the two types of bacteria, Wernegreen will be able to determine which genes the symbiotic bacteria have lost.
As the project progresses, Wernegreen also hopes to be able to make broader comparisons across a range of intracellular bacteria genomes. The ultimate goal of the project is to provide a theoretical framework that will help explain the evolutionary processes that have contributed to the diverse array of bacterial genomes structures and contents.
Wernegreens lab group is also examining the phylogenetic relationship between symbiotic bacteria and their insect hosts to determine whether these codependent species display congruent phylogenies. In other words, when the ancestral hosts evolved into a new species, did the ancestral symbiont species diverge as well, and vice versa? Basically they want to know whether the genetic family trees of host and symbiont branched at the same time. To answer this question, the group has developed molecular phylogenies for both the insect hosts and symbiotic bacteria.
Wernegreen says that the MBL has provided a unique community with expertise in molecular evolution and genomics. The Bay Paul Center brings together what she calls a critical mass of researchers who are using similar approaches but who have a variety of backgrounds and perspectives that strengthen the collaboration. The high throughput DNA technology available at the MBL has also been essential to the foundation of Wernegreens projects. With the advent of automated procedures, Wernegreen says that molecular geneticists can make a transition from trying to sequence a few genes to sequencing entire genomes. It allows us to ask questions on an entirely different scale. |